Steam Turbine Cycling
OPERATOR CONSIDERATIONS, BEST PRACTICES AND OPTIONS FOR OPTIMIZATION
Market dynamics are dictating increased unit cycling—are you ready?
By Thomas R. Reid, P.E., Manager of Engineering, TG Advisers, LLC. and John A. Moreci, MS, PMP, Principal Consultant, TG Advisers, LLC.
Regardless of age or design of steam turbines, there are industry best practices to help prepare for an uncertain future that certainly will include an uptick in cycling. In many cases, start-ups can be optimized from both a time and safety perspective with little or no capital investment through application of modern fracture mechanics technologies. A fascinating predicament is many steam turbines operating today were designed prior to the use of fracture mechanics principles in turbine generator design. Several of these units still follow the same startup guidance today as when they were new, 30+ years ago. With application of improved technical and analytical capabilities, as well as material testing that quantifies how rotors age, many units can take advantage of the margin inherent in most legacy designs. This article discusses the recommendations to assess feasibility for more aggressive cycling, engineering considerations that govern cold start procedures and common areas for optimization.
CYCLING FEASIBILITY ASSESSMENT
There are a number of potential conditions and operational limitations that can lessen the ability of a unit to cycle. TG Advisers recommends reviewing the more common items below as a first step feasibility assessment.
RECENT TURBINE INSPECTION DATA
Rotor Centerline Inspections: For bored rotors, a review of the most recent magnetic particle and ultrasonic bore inspection report is critical. These inspections define the location and size of detected flaws. If there are no reportable indications, a flaw equal to the minimum detectable flaw size in the location of peak stresses should be assumed. No bore rotors have lower centerline stresses. These rotors should be periodically ultrasonically inspected from the periphery. In all cases, findings should be assessed using a fracture mechanics approach.
Casing Condition: Casing cracking is common due to often large steam to metal temperature mismatches during startups. It is important to assess the depth of cracking relative to the wall or ligament thickness, as additional cycling will result in further crack propagation providing the stress field at the crack tip has not significantly reduced. Reducing steam to metal temperature mismatches during startup is the most effective way to reduce future cracking and propagation rates
Stationary and Rotating Blading Condition: Solid particle erosion rates in early rows of the HP and IP turbine will increase with additional cycling. On/off cycling causes exfoliation from the boiler, which carries over to the turbine. Depending on condition, stationary and rotating blading refurbishment or replacement may be required in advance of additional cycling.
Review of Rotor Properties: Rotors are supplied with Fracture Appearance Transition Temperatures (FATT) and Charpy Impact data that can be correlated to fracture toughness curves. Critical crack sizes can then be calculated from knowledge of the stresses, fracture toughness, and existing crack characteristics. Finding or obtaining this material data for a specific vintage rotor can be nearly an impossible task. However, opportunities may exist for material testing during outages to quantify missing material properties. In the absence of known material properties, conservative industry data can be applied from similar vintage forgings, although this can significantly hamper the optimization process.
REVIEW OF UNIT OPERATIONAL DATA
Differential Expansion: Adequate clearance is required between rotating and stationary parts and seals to ensure there is no contact during all modes of operation, including cold starts and full load trips. Rotors and casings heat differently and therefore grow at dissimilar rates (leading to differential expansion). Rotor length is also affected by speed. It is critical during outages that build clearances are restored to prior successful levels and rotor design float is maintained. For units inhibited by differential expansion, alarm levels should first be confirmed as being suitable based on build clearances. If appropriately set, operational sensitivity testing can be completed to determine the impact of different parameters, such as steam temperatures, on the differential expansion of the unit.
Vibration: Vibration can result for a multitude of reasons. One reason, particularly for older units, is a thermally sensitive rotor bow that develops with time due to asymmetric material properties. This bow acts as an unbalance force and can produce high vibration as the rotor passes through critical speeds. The characteristics of bows will also change with temperature. If vibration is of a high enough magnitude, it can have other detrimental impacts such as babbitt fatigue damage and radial rubbing of seals throughout the blade path.
Unit Metal Temperatures: If properly placed and calibrated, casing thermocouples can offer insight into the effectiveness and heating rates during startups. Although rotor metal temperatures will differ, review of this data can provide direction on adequacy of the existing start up processes.
Operational Transient Faults: With increased cycling, the probability of operational events such as water induction, overspeed, and loss of lube oil can increase. It is highly recommended procedures and protections against these events are understood and managed in advance of any cycling program.
The Optimization Process
Fatigue vs. Fracture Mechanics Approaches
Assuming there are no condition and operational limitations that significantly lessen the ability of a unit to cycle, an optimization study can be completed to evaluate the reduction of unit start time. Existing startup procedures and online stress monitors are designed to maximize the number of cycles before rotor bore crack initiation. The lower the bore stress levels during transients, the more cycles that can be expended before crack initiation occurs. Bore stresses cannot be directly measured so these models indirectly estimate stress levels based on metal temperatures and rotational speed. The most effective means to limit the stress levels in this scenario is to balance turbine speed and the rate of change of steam temperatures. This is achieved through extended start-up times. Thirty to forty years ago, fracture mechanics principals began to be routinely applied to steam turbine rotor design and life assessments. Rather than assuming a crack-free rotor and calculating an initiation time, a fracture mechanics approach assumes a flaw exists that may propagate based on a combination of cyclic stress cycles and material fracture toughness. In this case, rotor life is dictated by a safety factor on number of cycles for a crack to reach a critical size where rapid fracture would occur. Optimizing startup and load ramp times involves avoiding, to best advantage, combinations of high stress and low toughness.
High temperature rotors are more susceptible to failure when cold. New HP and IP rotors have Fracture Appearance Transition Temperatures (FATT) values of around 200°F. With service exposure, the FATT may degrade due to embrittlement in select temperature zones to above 300°F. Industry material test data for high temperature rotors shows the fracture toughness can improve with increased temperatures by a factor of approximately 3 to 5 during a cold start— this relationship is shown in Figure 2. For these reasons, high temperature rotor prewarming is necessary for safe operation.
It should be noted—low pressure turbine and generator rotors have superior fracture properties even at room temperature and do not limit the unit start-up rate. However, they may limit the ability to cycle the unit based on the size and locations of existing flaws.
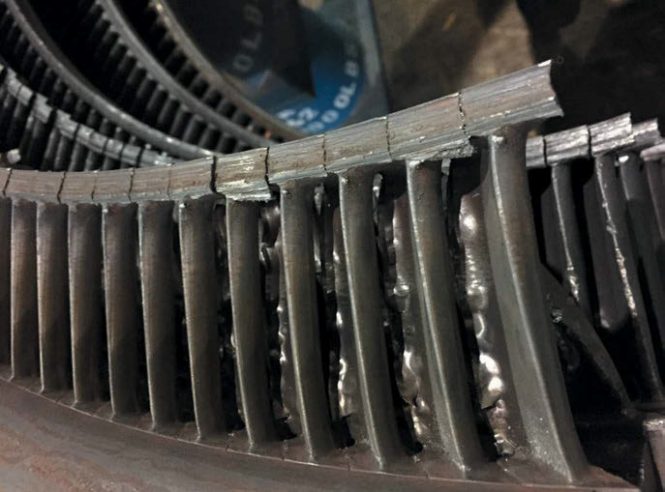
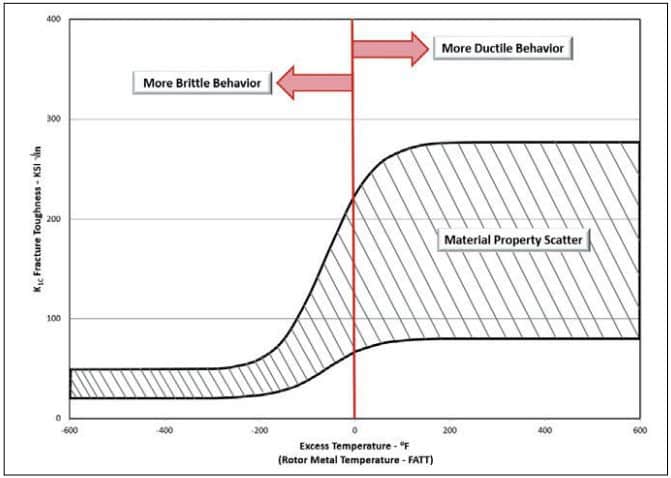
Analytical Approach to Optimization:
Rotor Temperature Model: Since fracture toughness is a strong function of temperature, and stresses are a function of both temperature and speed, rotor temperature is a critically important parameter. An optimization of start-up and load ramp times must include a transient heat transfer analysis. A snapshot of a temperature distribution for an IP-LP rotor from such an analysis is shown in Figure 3. This model utilizes unit operational data to calculate the heating throughout start up.
Rotor Stress Model: Similar to the thermal analysis, stress distributions are calculated along the rotor throughout the start up as well. Figure 4 shows a snapshot of stress distributions on the same IP-LP rotor, which illustrates that rotor body stresses are greatest at the bore surface.
Resulting Critical Crack Sizes: Limiting critical crack sizes can then be calculated throughout the start up from knowledge of the temperature and fracture toughness relationship, stresses and existing flaws in the rotor. The calculated critical crack sizes are compared to known flaws in the rotor, to ensure substantial safety margin. Figure 5 shows calculated critical crack sizes at the bore beneath each stage of the IP rotor. As can be seen, the limiting portion on this start up (smallest calculated critical crack size) occurs within 1.5 hours of turbine roll. It is important to be conservative with the introduction of stresses during cold starts, particularly while the rotor is at or below the FATT. However, from a rotor integrity perspective, there is little technical benefit to prolonging start up processes once good fracture properties have been achieved.
Target Areas for Optimization
If there are no major condition or operational red flags and the baseline analysis is favorable, then optimization can be considered. The following are common areas that should be considered in this process. As a first step, benchmarking of current start up conditions and turbine roll parameters is essential. Ensure critical OEM requirements are met, such as steam to metal temperature differentials and superheat requirements.
Turbine Roll Conditions: There are a number of permissives that must be met prior to rolling a steam turbine off gear. Some examples are steam line warming, steam superheat requirements and rotor eccentricity. Note rolling at optimal steam pressures and temperatures can greatly improve cycle time. Consider rolling at lower steam pressures, which can improve superheat levels and lower the steam to metal temperature mismatch. However, steam temperatures must be well above the rotor metal FATT or there will be little heating benefit along with a risk of exposing the rotor to high rotational stresses while it has poor fracture properties.
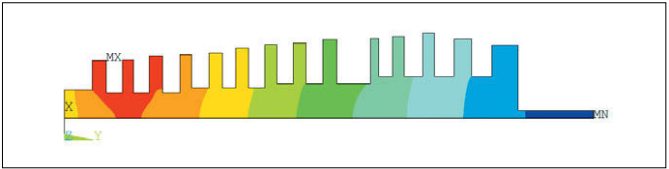
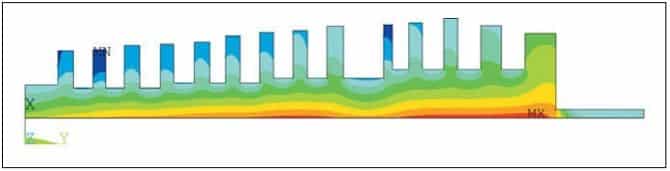
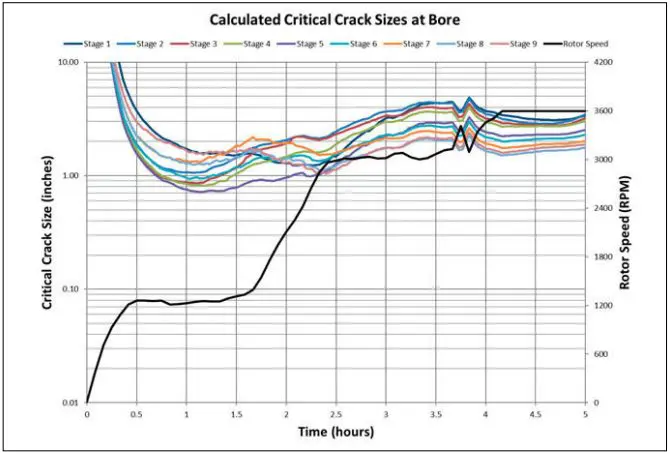
Optimization of Hold Speeds and Times: A common and potentially concerning finding are cold start procedures that do not include a low speed heat soak. As previously mentioned, the objective is to hold the rotor at speeds well below operating speed to minimize centrifugal stresses until the rotor has good fracture toughness. Ramping the unit to high speeds with a cold rotor can have significant consequences and is not recommended by TG Advisers. Adding or extending a low speed hold can improve the safety margin of the startup significantly, and often allow for reductions on the later stages of start up when the material exhibits good fracture properties. Thermal stresses are controlled by maintaining steam to metal mismatch at reasonable levels; a good target is less than 250°F but lower is better.
It is important to note hold speeds must not be arbitrarily selected. In some cases, TGA has found hold speeds have been modified because of lateral vibration concerns. This can be a dangerous practice since holding at a non-compliant speed can cause LP blading to vibrate at its natural frequencies, which would not be known to an operator. Blade vibration cannot be measured by bearing lateral vibration probes. Turbine instruction manuals will include allowable hold speeds or avoidance zones. Ensure these are adhered to and are still current if a retrofit or a new LP blade design was installed.
Reduction in Full Speed No- or Low-Load Holds: It is generally accepted that high temperature rotor fracture toughness curves reach an upper shelf, after which there is no appreciable improvement in rotor properties with additional heating. Further, there are other concerns that can occur at full speed low-load operation. Some examples are windage heating, stall flutter vibration of last stage LP blades, last stage trailing edge blade erosion, and increased differential expansion.
Opportunities for Pre-Heat: If an impending startup is known in advance, an auxiliary boiler or adjacent unit steam source can be used in most cases to supply steam to the glands while the unit is on turning gear. A benefit of this approach is the glands are located at the exhaust ends of high temperature rotors where thermal mass and stresses are greatest. Since these locations will heat the slowest during a start-up, significant benefit can be realized in startup time margin and reduction.
RESULTS OF A RECENT OPTIMIZATION
Table 1 summarizes the reduction in cold start-up time for a recently evaluated vintage unit achieved by applying finite element and fracture mechanics analyses and industry best practices. In this particular case, the operator is now able to dispatch the unit in one-third less time than before the optimization.
CLOSING COMMENTS
We are in the midst of a challenging and dynamic time in the industry, where flexibility is a significant benefit. Utilizing sound engineering practices, inspection results and modern fracture mechanics principals offers an opportunity to optimize steam turbine startup times. In TG Advisers’ experience, cold start times on older steam turbines commonly can be reduced by 25% to 50% while still adhering to critical OEM design parameters and requirements. These reductions provide significant startup cost fuel savings and enhance an older steam unit’s ability to be dispatched in today’s challenging environment.
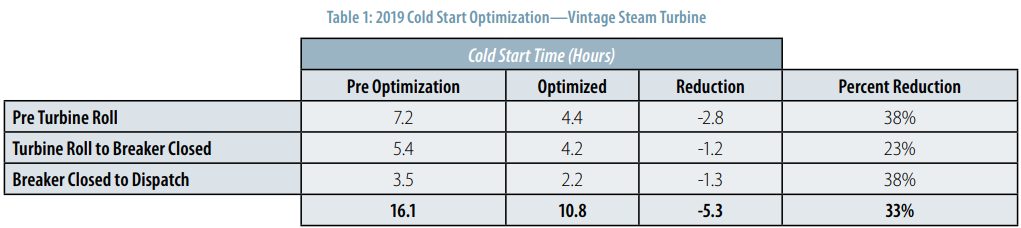